Overview
- Ploidy is the number of sets of homologous chromosomes in an organism.
- The common domesticated potatoes are tetraploid, with four sets of homologous chromosomes.
- Lesser known domesticated potatoes may also be diploid (two sets), triploid (three sets), or pentaploid (five sets).
- Wild potatoes can range in ploidy from diploid (two sets) to hexaploid (six sets).
- Endosperm Balance Number (EBN) is an experimentally determined score that can be used to predict the success of crosses between potatoes of differing ploidy or species.
- Only potatoes with the same EBN are normally able to form viable seed when crossed.
- In Solanum tuberosum potatoes, EBN is equal to ploidy; diploids are 2EBN and tetraploids are 4EBN.
- Potatoes sometimes produce unreduced gametes, which have double the effective EBN and ploidy, allowing crosses between diploids and tetraploids.
- Although the mechanism is not well understood, and contradicts the expected result based on EBN, triploids sometimes result from crosses between tetraploid and diploid domesticated potatoes.
- Potato EBN is probably controlled by alleles on two different chromosomes that are additive.
- Potato ploidy can be determined with a microscope, either by counting guard cell chloroplasts, or by directly counting chromosomes.
Introduction
Once you move past breeding only with domesticated tetraploid potatoes, it becomes helpful to understand the crossing barriers between different levels of ploidy and between domesticated and wild species. Different levels of ploidy are a factor in breeding many species, but, in potatoes, ploidy interacts with an additional factor: the endosperm balance number (EBN). Together, ploidy and EBN can be used to predict which crosses can be successful. It will take a little work to understand all of this, particularly if you don’t have much foundation in biology. That said, there is nothing here that you need a fancy education to understand. Just take your time. This is a jargon-heavy topic, with more than one way to express many of the ideas. I have done my best to de-jargonize where possible, but there is quite a bit of terminology that you will need to absorb if you want to proceed from here to reading the scientific literature.
How Seeds are Formed
Before we get down into the details of potato ploidy, it is important to review the process by which seeds are formed in flowering plants. Some of this will be immediately relevant and some of it will be more important later on. It might not be the information that you are looking for right now, but understanding it will pay off a few pages down.
All plants undergo alternation of generations, a sporophyte generation and a gametophyte generation. The sporophyte is what we recognize as the plant and the gametophytes are the male and female components contained within flowers. When you look at a potato plant growing in your garden, you are looking at the sporophyte. The sporophyte produces spores, which in turn produce gametophytes via meiosis, a process that doubles a cell while halving the number of sets of chromosomes. The gametophytes have half as many sets of chromosomes as the sporophyte does; they are haploid. The gametophytes produce gametes: ovules and pollen. The gametes are produced by mitosis, a process that doubles a cell while maintaining the same number of chromosomes. Because the gametophytes are haploid and the same number of chromosomes is maintained by mitosis, the gametes are also haploid.
Flowering plants, like potatoes, undergo double fertilization. This is the part that will be particularly important later. When a grain of pollen lands on a pistil, it germinates and then produces a pollen tube that grows down into the ovary where it connects to an ovule and introduces two haploid sperm. One of the haploid sperm unites with a haploid egg and develops into a diploid zygote. The zygote will go on to become the embryo if the seed develops successfully. But what about the second sperm? It joins with a structure known as the central cell that contains two polar nuclei. The polar nuclei are haploid, but there are twice as many as in a normal cell. Most cells have one nucleus that contains the chromosomes. Because the central cell has two nuclei, it has two sets of chromosomes. When the central cell is joined with the second sperm, it then has three sets of chromosomes; it is triploid. This triploid cell becomes the endosperm, the source of nutrition for the embryo in the seed. Because it is the result of a separate fertilization, the endosperm is technically a separate organism from the embryo, but its only real purpose is to supply a source of energy for the embryo.
The most important part to understand here is that the fertilization of a flower results in the creation of both an embryo and an endosperm and that the endosperm has 50% more chromosomes than the embryo. Both the embryo and the endosperm must be viable in order for the seed to finish its development. The successful formation of the endosperm is one of the main limiting factors in potato crosses.
Domesticated and Wild Potatoes
The main domesticated potato is Solanum tuberosum, which comes in both diploid and tetraploid forms (more on that below). There are also some lesser domesticated potatoes that most people outside of South America have never encountered, including S. juzepczukii, S. curtilobum, and S. ajanhuiri. In some places, particularly in South America, domesticated potatoes frequently escape cultivation. We generally describe those as feral potatoes. Wild potatoes, on the other hand, are truly wild and generally grow without any human intervention. There are about 100 recognized species of wild potatoes, ranging from the southwest United States all the way down to the bottom of South America, with the greatest density in the Andes. Wild potatoes are a valuable source of genes for breeding work with the domesticated potato and one of the benefits of understanding more about potato ploidy is learning how to make crosses between domesticated and wild species.
Ploidy
Like many plants, potatoes have several possible levels of ploidy. Ploidy is the number of sets of homologous chromosomes in an organism. Homologous in this case means roughly “matching.” The chromosomes are not identical, but they contain the same genes. The values of those genes, the alleles, are often different. Humans are diploid. We have two sets of homologous chromosomes; one copy is inherited from each of our parents. In many organisms, more than one set is inherited from each parent. These organisms are called polyploids, because they have more than two sets of homologous chromosomes. The common potatoes that you will find in grocery stores in North America and Europe are polyploids and, more specifically, tetraploids. They have four sets of homologous chromosomes, with two inherited from each parent. In South America, they also have many diploid potatoes. Only diploids and tetraploids are commonly found in the domesticated potato (Solanum tuberosum), but there are rarer levels of ploidy, including triploids and pentaploids. Some levels are rarely or never found in nature but are possible with human intervention, including monoploids and octoploids.
Common Levels of Ploidy in Potatoes
Level | Chromosome Sets | Chromosomes (2n) |
x | n | Equation | Examples |
Monoploid | 1 | 12 | 12 | * | x = 12 | Monoploids can only be created in laboratories. (For example, by culturing the pollen of diploid potatoes.) They are used for breeding, particularly attempting to produce homozygous diploid potatoes. |
Diploid | 2 | 24 | 12 | 12 | 2n = 2x =24 | Diploids are very common among domesticated and wild potatoes in South America. |
Triploid | 3 | 36 | 12 | 18** | 2n = 3x = 36 | Triploids are typically not the main level of ploidy in a potato species, but result from inter-species crosses or from mutations. S. juzepczukii, a domesticated bitter potato from the Andes is triploid. |
Tetraploid | 4 | 48 | 12 | 24 | 2n = 4x = 48 | Almost all domesticated potatoes in North America, Europe, and Chile and many from the Andes are tetraploid. |
Pentaploid | 5 | 60 | 12 | 30** | 2n = 5x = 60 | Pentaploids are typically not the main level of ploidy in a potato species, but result from inter-species crosses. S. curtilobum, a domesticated bitter potato from the Andes is pentaploid. |
Hexaploid | 6 | 72 | 12 | 36 | 2n = 6x = 72 | Some wild and/or marginally domesticated potatoes like Solanum demissum are hexaploids. |
* A monoploid cannot undergo further division, so it has no haploid number (n).
** Triploids and pentaploids generally don’t undergo normal meiosis and the actual number of chromosomes can vary.
Monoploid and Haploid Numbers
When describing the genome of a species, we use the monoploid number (x) and the haploid number (n) to show the relationship between the number of unique chromosomes in that species (x) and the total number of chromosomes present in its gametes (n).
x is the monoploid number, the number of chromosomes that are unique in an organism. It is the minimum number of chromosomes that could produce a viable organism of that species. In the case of potatoes, x = 12. When additional sets of chromosomes exist, they are termed homologous. A diploid potato has two homologous copies of each chromosome.
n is the haploid number, the number of chromosomes found in gametes (ovules and pollen), which are normally haploid. In the case of potatoes, n is some multiple of 12.
Let’s do a couple of examples to get the hang of this:
A diploid potato has 2 sets of chromosomes, for a total of 24. x = 12 because 12 will always be the monoploid number for potatoes. This doesn’t change. n = 12 because the haploid form of a diploid has half the number of sets of chromosomes, which is one. The haploid form of a diploid is monoploid. The haploid number equals the monoploid number. n = x so it is also true that 2n = 2x and this is how we describe the genome of a diploid potato: 2n = 2x = 24. A diploid potato has 24 chromosomes, which is twice the monoploid number and twice the haploid number.
A tetraploid potato has 4 sets of chromosomes for a total of 48. x = 12 because 12 will always be the monoploid number for potatoes. This doesn’t change. n = 24 because the haploid form of a tetraploid has half the number of sets of chromosomes, which is two. The haploid form of a tetraploid is diploid. The haploid number is twice the monoploid number. n = 2x so it is also true that 2n = 4x and this is how we describe the genome of a tetraploid potato: 2n = 4x = 48. A tetraploid potato has 48 chromosomes, which is four times the monoploid number and twice the haploid number.
Now let’s do an odd one. A triploid potato, like Solanum juzepczukii, has 3 sets of chromosomes for a total of 36. x = 12 because 12 will always be the monoploid number for potatoes. n = 18, half the number of chromosomes. 18 is a strange number though, because this is 1.5 sets of chromosomes. n = 1.5x and 2n = 3x. For an organism to have normal sexual reproduction, n must be a whole number. In this case, n is not a whole number, so Solanum juzepczukii has impaired sexual reproduction. We’ll get into the details futher down.
Haploids
Haploid is a general term that always describes half (n) of the sporophytic chromosome number (2n), regardless of the level of ploidy of the plant. The gametes of a diploid, a tetraploid, or an octoploid can all be correctly described as haploid, but there are also more specific terms. The haploid of a tetraploid can be described as a dihaploid, that is, a haploid that is diploid. Similarly, the haploid of a hexaploid can be termed a trihaploid and the haploid of an octoploid can be termed a tetrahaploid. These terms aren’t necessary, but sometimes add clarity and you are likely to run into them in reading more advanced texts.
Haploids are not always gametes. It is possible to create plants that are haploids in the sporophyte stage. Haploid potato plants have half of the normal number of sets of chromosomes. They are commonly used in tetraploid potato breeding to facilitate crosses at the diploid level. A typical scenario involves producing a dihaploid from a tetraploid (the haploid is a diploid, since it has half of the chromosomes of a tetraploid), then crossing it with a diploid, selecting progeny from the cross, and then restoring them to tetraploids by chemical doubling. One common way to produce haploid potatoes is to culture the pollen or the ovules, which grow into plants that have only half the genetic material of the parent because they haven’t been united with another gamete.
Interactions of Ploidy in Crosses
Ploidy imposes some constraints on compatibility between varieties. In general, the domesticated potatoes are compatible for breeding when they have the same level of ploidy. Cross a diploid with a diploid and you get a diploid; cross a tetraploid with a tetraploid and you get a tetraploid. The situation is more complex when the number of chromosome sets is an odd number or when you try to make crosses between different levels of ploidy.
Potatoes with odd levels of ploidy, like triploids and pentaploids, generally cannot be crossed at the same level because they don’t produce a full set of chromosomes when divided in half. When a cell with an odd numbered ploidy divides by meiosis, you don’t get an even division of chromosomes. With a triploid, you would expect to get one and a half sets of chromosomes in each gamete, with no way to guarantee that the chromosomes in the half set are homologous with any other randomly chosen half set. In reality, that is a major simplification and the way that single set of chromosomes gets divided is even less predictable. Because of this, triploids and pentaploids tend to be sterile, or at least dysfunctional and difficult to cross. Interestingly, they can be more easily crossed to potatoes with even levels of ploidy than to each other. Such crosses tend to produce aneuploids – varieties that have an irregular number of chromosomes. For example, crossing a pentaploid with a tetraploid usually yields a near-tetraploid aneuploid. A common result would be a tetraploid that has six extra chromosomes. That plant will often be self compatible and future generations will tend to lose the extra chromosomes, eventually becoming true tetraploids.
It is possible to get whole number gametes from a potato with odd ploidy, but the odds are (1/2)^(x-1). In the potato, with x=12, that means the odds of getting a whole number gamete are about 0.05% or roughly 1 in 2000. That is not completely unworkable for pollen, since it is produced in such large amounts, but it will likely require quite a bit of persistence to make a successful cross.
Among domesticated potatoes, it is possible to make crosses between different levels of ploidy, but this is not entirely straightforward. In any cross between a diploid and a tetraploid, you would end up with a gamete from the diploid that has 1 set of chromosomes and a gamete from the tetraploid that has two sets of chromosomes. Combine these and you would predict a triploid zygote with 3 sets. In fact, this rarely happens due to what was once commonly known as the “triploid block,” a consequence of failure to develop a viable endosperm.
Unreduced (2n) Gametes
As we have discussed in the previous sections, meiosis normally produces haploid (1n) gametes. The fusion of two 1n gametes results in a 2n organism. In many plants, and particularly in the potato, 2n gametes may also be produced on occasion. The overall frequency of 2n gamete production in Solanum species has been estimated to fall between 1 and 40%, depending on the specific mutations present. These gametes are termed “unreduced” because they have not undergone the meiotic division that results in the normal 1n gamete. As you can imagine, a 2n gamete would be problematic in a cross between two varieties with the same level of ploidy. If one parent produces a normal 1n, 1x gamete and the other produces an unreduced 2n, 2x gamete, the theoretical outcome is a 2n, 3x (triploid) zygote. In any case where the division of x by n does not result in a whole number, meiosis will be irregular at best.
On the other hand, consider the cross of a tetraploid potato and a diploid potato that produces 2n gametes. In this case, the tetraploid normally produces a 1n, 2x gamete after reduction. If the diploid produces an unreduced (2n, 2x) gamete, their fusion will result in a normal tetraploid (2n, 4x). This is the means by which diploid potatoes can be crossed to tetraploids.
Unreduced pollen and ovules are both possible, but 2n pollen is much more common simply because much more pollen is produced. Because of this, obtaining the pollen from the parent with the lower ploidy level is much more likely to be successful. That is, when crossing tetraploids and diploids, you generally want to produce a tetraploid x diploid cross, rather than diploid x tetraploid. Both are possible, but the odds of success with the first are much greater.
The following table summarizes the possible outcomes. Green rows are commonly used in potato breeding. Red rows are usually not possible due to endosperm problems. Yellow rows are possible, but very low probability events.
Cross | Chromosome Sets in Female Gamete | Chromosome Sets in Male Gamete | Chromosome Sets in Zygote | Ploidy | Notes |
Tetraploid (reduced) x Tetraploid (reduced) | 2 | 2 | 4 | Tetraploid | This is the normal case for tetraploid crosses. |
Tetraploid (reduced) x Tetraploid (unreduced) | 2 | 4 | 6 | Hexaploid | Blocked |
Tetraploid (unreduced) x Tetraploid (unreduced) | 4 | 4 | 8 | Octoploid | Rare. The odds of getting two unreduced gametes are very low. The genes involved are uncommon and recessive. Getting a homozygous recessive configuration is difficult in tetraploids due to tetrasomic inheritance. |
Diploid (reduced) x Diploid (reduced) | 1 | 1 | 2 | Diploid | This is the normal case for diploid crosses. |
Diploid (reduced) x Diploid (unreduced) | 1 | 2 | 3 | Triploid | Blocked |
Diploid (unreduced) x Diploid (unreduced) | 2 | 2 | 4 | Tetraploid | Rare. The odds of getting two unreduced gametes are very low. |
Tetraploid (reduced) x Diploid (reduced) | 2 | 1 | 3 | Triploid | Blocked. However, sometimes this works for other reasons.* |
Tetraploid (reduced) x Diploid (unreduced) | 2 | 2 | 4 | Tetraploid | This is usually uncommon, but this is the most direct way of crossing diploids to tetraploids.** |
Tetraploid (unreduced) x Diploid (unreduced) | 4 | 2 | 6 | Hexaploid | Blocked |
Diploid (reduced) x Tetraploid (reduced) | 1 | 2 | 3 | Triploid | Blocked (but not always***) |
Diploid (reduced) x Tetraploid (unreduced) | 1 | 4 | 5 | Pentaploid | Blocked |
Diploid (unreduced) x Tetraploid (unreduced) | 2 | 4 | 6 | Hexaploid | Blocked |
* In some cases, diploid pollen induces parthenogenesis in a tetraploid. This results in a diploid (dihaploid) which only has genetic contribution from the female parent. Some varieties of phureja type diploids, known as haploid inducers, are used to produce dihaploids for breeding.
** The alternative is to convert tetraploids into dihaploids by anther or ovule culture and then to make crosses at the diploid level. This requires more work up front and requires some additional technical expertise, but is much more efficient for large scale work. Once you have produced the dihaploid, it can then be grown out like any other potato to make lots of crosses. *** For reasons that are not entirely understood, but that may involve mitosis without cellular division in the central cell, sometimes this cross produces viable triploids. |
Endosperm Ratio
If you skipped the introduction “How Seeds are Formed,” now would be a good time to read it. The endosperm balance number theory adds a twist to understanding how potatoes of different ploidy levels can be crossed. Many crosses that appear to be possible based purely on ploidy do not succeed. They don’t succeed because the endosperm does not develop correctly, which produces nonviable seed. The reasons for this are not completely understood, but EBN is generally able to predict which crosses will succeed even if we don’t know exactly why.
Before the endosperm balance number theory was developed, there was a simpler theory that proposed that there must be a 2:1 female:male ratio of chromosome sets in the endosperm in order for it to develop properly. As we discussed at the beginning, the endosperm is created through the fusion of the two 1n nuclei of the central cell (giving 2n total) and the 1n nucleus of the sperm, which produces a triploid endosperm. That 2x:1x relationship is essential to producing a viable endosperm. While the most common case is a triploid (2x:1x) endosperm, there is more than one way to obtain the 2:1 ratio, including hexaploids (4x:2x) and dodecaploids (8x:4x).
Let’s revisit the ploidy crossing chart from above, but look at the endosperm ratios instead and you will see how this pattern is consistent with the blocked combinations:
Cross | Chromosome Sets in Female Gamete | Chromosome Sets in Central Cell |
Chromosome Sets in Male Gamete |
CC:Male Ratio | Outcome |
Tetraploid (reduced) x Tetraploid (reduced) | 2 | 4 | 2 | 2:1 (Hexaploid) |
Success |
Tetraploid (reduced) x Tetraploid (unreduced) | 2 | 4 | 4 | 1:1 | Failure |
Tetraploid (unreduced) x Tetraploid (unreduced) | 4 | 8 | 4 | 2:1 (Dodecaploid) |
Success (but almost impossible for other reasons) |
Diploid (reduced) x Diploid (reduced) | 1 | 2 | 1 | 2:1 (Triploid) |
Success |
Diploid (reduced) x Diploid (unreduced) | 1 | 2 | 2 | 1:1 | Failure |
Diploid (unreduced) x Diploid (unreduced) | 2 | 4 | 2 | 2:1 (Hexaploid) |
Success (but uncommon) |
Tetraploid (reduced) x Diploid (reduced) | 2 | 4 | 1 | 4:1 | Failure |
Tetraploid (reduced) x Diploid (unreduced) | 2 | 4 | 2 | 2:1 (Hexaploid) |
Success |
Tetraploid (unreduced) x Diploid (unreduced) | 4 | 8 | 2 | 4:1 | Failure |
Diploid (reduced) x Tetraploid (reduced) | 1 | 2 | 2 | 1:1 | Failure |
Diploid (reduced) x Tetraploid (unreduced) | 1 | 2 | 4 | 1:2 | Failure |
Diploid (unreduced) x Tetraploid (unreduced) | 2 | 4 | 4 | 1:1 | Failure |
Endosperm Balance Number
The endosperm ratio is wonderful; it neatly explains why crosses succeed or fail and it would be great if that closed the book on potato crosses. Unfortunately, it isn’t a complete explanation. There are exceptions. Crosses with odd ploidies and with certain related Solanum species don’t always work as we would predict based only upon the endosperm ratio. The Endosperm Balance Number was conceived to fill in the gaps. Endosperm balance numbers range from 1 to 4 and are experimentally determined, because we don’t really know the genetic basis of this feature yet. Any two species with the same EBN can be crossed. EBN is also sometimes referred to as a “crossability group,” using the same numbers. So, 4EBN is crossability group 4, 2EBN is CG2, etc. The following table summarizes some EBN assignments for common species and you can find many more in the scientific literature:
Species | Ploidy | EBN |
Domesticated Potatoes | ||
S. tuberosum | Diploid | 2 |
S. tuberosum | Tetraploid | 4 |
S. curtilobum | Pentaploid | 4 |
S. juzepczukii | Triploid | 2 |
Wild Potatoes | ||
S. acaule | Tetraploid | 2 |
S. boliviense | Diploid | 2 |
S. brevicaule | Diploid | 2 |
S. cardiophyllum | Diploid | 1 |
S. chacoense | Diploid | 2 |
S. commersonii | Diploid | 1 |
S. demissum | Hexaploid | 4 |
S. stoloniferum | Tetraploid | 2 |
S. verrucosum | Diploid | 2 |
You can see a more complete list of wild potato species with ploidy and EBN here.
In many of the familiar cases, the ploidy and the EBN match. Diploid S. tuberosum is 2EBN, while tetraploid S. tuberosum is 4EBN. No surprises there, but S. acaule is a tetraploid with 2EBN, meaning that it can be more easily crossed with diploids than tetraploids. Similarly, the hexaploid S. demissum with 4EBN can be crossed to the tetraploid S. tuberosum, which is also 4EBN.
Unlike the endosperm ratio, EBN predicts the successful production of triploids and pentaploids from certain crosses. For example, S. juzepczukii, which is triploid, is thought to have resulted from a cross between tetraploid S. acaule and diploid S. tuberosum. Both of these species have an EBN of 2, indicating that they are compatible, but since one is tetraploid and the other is diploid, a cross involves the fusion of a 2x gamete and a 1x gamete, resulting in a 3x (triploid) hybrid.
If you have been paying attention, you might be wondering how unreduced (2n) gametes fit into this system. Just double the EBN. Therefore, although diploid S. tuberosum is 2EBN, its unreduced gametes have an effective EBN of 4, which explains how the tetraploid x diploid (unreduced) cross fits into the EBN system. You can use EBN to plan chains of crosses to move genes from one level to another, either by relying upon unreduced gametes or by chemically doubling the genome of the lower EBN species (e.g. with colchicine or oryzalin).
(I knew that someone would ask and it took almost no time at all. There is a 3EBN level, although it doesn’t appear in the chart. As far as I know, there are no naturally occurring species of 3EBN, but there are laboratory-created somatic hybrids between Solanum species that are 3EBN.)
It may take some time to digest all of this, but with an understanding of potato ploidy and EBN, you can maximize seed production in relatively simple crosses between different levels of S. tuberosum or plan complex crosses between multiple wild species to bridge genes into the domesticated potato.
The Potato Gene Pool
Yet another way of classifying potatoes is in terms of the degree of separation between a given species and the domesticated potato. Harlan (1971) proposed dividing crops and their wild relatives into primary, secondary, and tertiary gene pools. The primary gene pool consists of the domesticated species and others that interbreed freely and could be considered all one species (even if they are actually divided). The secondary gene pool consists of species that can interbreed with the primary gene pool, but with limitations that affect viability or fertility. The tertiary gene pool consists of species that generally cannot directly interbreed with the primary gene pool (or do so with extreme limitations) without extraordinary intervention like ploidy manipulation or bridging through crosses with the secondary gene pool.
For potato, the primary gene pool is comprised of those species that will cross readily with the domesticated tetraploid potato. This includes all 4EBN species and many South American 2EBN species. The secondary gene pool includes mostly 2EBN species and the tertiary gene pool mostly 1EBN species.
Triploid Domesticated Potatoes
I just spent a lot of time explaining why domesticated triploid potatoes are unlikely, but they do exist. There is a fairly large group of domesticated Andean potatoes known as the chaucha group, or Solanum x chaucha, depending on what system of classification you prefer. And triploids have been observed with some frequency in tetraploid x diploid crosses for many years. In fact, in some crosses, the rate of triploid formation may be as high as 74.4% (Graebner 2019). So, what’s going on here? I don’t think that there is a satisfying answer yet. Graebner suggested that they may have been successful in germinating seeds that lacked an endosperm. They extracted seeds from berries by hand and such seeds would typically be floated off in bulk seed cleaning, rejected at sowing due to malformed appearance, or simply unsuccessful at forming seedlings due to insufficient care. It is possible that they were lucky in their parent selection as well. Fractional EBNs might be possible, in which case the parents might have had non-integer EBNs, leading to unusual compatibilities.
The Genetics of EBN
The genetics controlling EBN have not been definitively established, but there are several proposed models. The simplest is a single dosage-dependent, multiallelic gene with values of 1/2 or 1 EBN that is always homozygous within a given genome. Unfortunately, this model does not account for the outcomes of many crosses, particularly wide crosses between different species. A better model is proposed by Camadro (1994), involving two separate loci on two different chromosomes, with allele values of either 0 or 1/2 EBN, which are typically homozygous:
EBN Allele at First Locus | EBN Allele at Second Locus | Ploidy | Effective EBN | |
2x(1EBN) | 1/2 | 0 | 2x | ((1/2 + 0) * 2) = 1 |
4x(2EBN) | 1/2 | 0 | 4x | ((1/2 + 0) * 4) = 2 |
2x(2EBN) | 1/2 | 1/2 | 2x | ((1/2 + 1/2) * 2) = 2 |
4x(4EBN) | 1/2 | 1/2 | 4x | ((1/2 + 1/2) * 4) = 4 |
6x(4EBN) | 1/2 (2) + 1/2 (4) | 1/2 (2) + 0 (4) | 6x | ((1/2 + 1/2) * 2) + ((1/2 + 0) * 4) = 4 |
The rest of what follows in this section considers exceptions and unusual cases for EBN and may not enhance your understanding if you are not working with wild potatoes or interspecies hybrids.
We can associate the alleles fairly neatly with the three potato genomes. The A genome is 1/2:1/2, the B genome is 1/2:0, and the P genome is a mix, with about two thirds of P genome species having 1/2:1/2 and the remaining third 1/2:0. There do appear to be some species that don’t fit though, particularly among the hexaploid species. An ABP hexaploid would be expected to have an EBN of (2*(1/2+1/2))+(2*(1/2+0))+(2*(1/2+1/2)) = 5EBN or (2*(1/2+1/2))+(2*(1/2+0))+(2*(1/2+0)) = 4EBN. All tested ABP hexaploids have 4EBN. There are also A genome hexaploids, like S. demissum. An all A genome hexaploid would be expected to have 6*(1/2+1/2) = 6 EBN, but they have been reported to have 4EBN. Also, tetraploid S. acaule, which bears only the A genome, is 2EBN, which would require a configuration of 4*(1/2+0). This only makes sense if such species have copies of the A genome that are 1/2:0. So, there is probably more allelic diversity within the three potato genomes than it seems at first and there appears to be something usual about the A genome found in some polyploid species.
This model has other interesting implications. For example, a domesticated triploid would actually be 3EBN ((1/2 + 1/2) * 3). In reality, the gametes of a species with odd ploidy will vary in chromosome number because the extra set of chromosomes can’t pair and gets allocated unpredictably. The gametes of a triploid will not be regularly 1.5-ploid as you would expect if the chromosomes divided equally. Instead, the gametes will range between monoploid and diploid and not all will contain the chromosomes involved in EBN. A gamete from a triploid that is monoploid or near monoploid will likely have an EBN of 1, while a diploid or near diploid gamete is more likely to have an EBN of 2. A gamete with 13 chromosomes (monoploid + 1 extra) could not be 2EBN because it could only have three EBN chromosomes. It could be 1.5EBN if the one extra chromosome has a 1/2 EBN allele, but the odds favor the extra chromosome not being an EBN chromosome (there is a 2 in 12 chance of getting an EBN chromosome), in which case it would be 1EBN. A gamete with 14 chromosomes (monoploid + 2) could be 2EBN, although the odds of getting the two EBN chromosomes from a set of 12 is 1 in 144, so it is unlikely.
You can also get gametes with irregular EBN from hybrids or potentially from wild species that don’t pair evenly in meiosis. In multi-genome hybrids, chromosomes tend to clump together with other chromosomes from their own genome, producing unbalanced gametes. A mixed genome 2EBN tetraploid has eight chromosomes bearing either 1/2 or 0 EBN alleles. If those chromosomes segregate irregularly, there is a possibility of getting gametes of 1 (expected), 1.5, 0.5, 2, or 0 EBN, depending on which chromosomes end up in the gamete. So, after fusing with a normal gamete, there is potential for 2EBN, 2.5EBN, 1.5EBN, 3EBN, and 1EBN progeny. These are not all equal in probability. 1EBN gametes are by far the most likely result, but the others are at least theoretically possible.
Manipulating Ploidy
Ploidy (and, by extension, EBN) can be manipulated in order to make crosses that are otherwise impossible. Ploidy can be doubled (or occasionally multiplied to even higher levels) through the use of mitotic inhibitors. Three mitotic inhibitors are commonly used: colchicine, oryzalin, and amiprophos-methyl. Colchicine has been in use the longest and is, therefore, the most widely known, but it is a relatively dangerous chemical that hobbyists cannot easily obtain. The alternatives, oryzalin and amiprophos-methyl are safer and more widely available, as components of common herbicides. Oryzalin is generally considered to be the most effective agent for doubling potatoes, followed by amiprophos-methyl. Both can be purchased in pure form from many laboratory supply companies. Soaking potato shoots in 25-30 micromolar solutions of Oryzalin for 24 hours should produce some doubled plants. More specific recommendations can be found in the research literature. I do not give specific instructions here so that you will do your own research and familiarize yourself with the safety of these compounds and the procedures for using them.
Ploidy can also be halved, producing haploids. Dihaploids, produced by halving tetraploid varieties, are commonly used in order to make crosses at the diploid level. The most reliable means of halving is pollen culture, where pollen or anther tissues are grown on tissue culture medium to produce plants with only the male genetic contribution. This is a complicated procedure beyond the scope of this overview, but if you have the experience and equipment to do plant tissue culture, you should be able to read the literature to obtain the necessary details. Luckily, there is an easier method, known as haploid induction.
Some domesticated diploid potatoes are able to produce 2n pollen that, for reasons that are not yet completely understood, can pollinate tetraploid potatoes without contributing any chromosomes. The result is that the tetraploid so pollinated will produce diploid seeds. Varieties that have proven to do this reliably are known as “haploid inducers,” but any diploid potato that produces a lot of 2n pollen is likely to produce some haploids in crosses with the tetraploid domesticated potato. The most recent evidence indicates that the mechanism by which this works is most likely genome elimination, where the chromosomes delivered by the haploid inducer are lost early in cell division. In fact, some amount of the haploid inducer genetics make it into the haploid in about 10% of cases. While it is easy to make crosses with haploid inducers, many of the progeny will be normal tetraploids or even triploids, so a considerable amount of screening is involved to determine which seedlings are truly dihaploid. Even with a haploid inducer, it will be a big job to make the crosses and identify any haploid progeny that have good performance. Most hobbyists would probably be better off obtaining haploid tuberosum varieties from genebanks, which have already been screened and selected.
Four haploid inducers are widely available from potato genebanks: IVP35 (CIP 800951/PI 584995), IVP48 (PI 584993), IVP101 (CIP 801037/PI 584994), and PL4 (CIP 596131.4/PI 695419). Others may be available regionally. Most haploid inducers have the seed spot (Bc) gene, which enables you to screen out any seeds that show a seed spot, since those indicate an unwanted genetic contribution from the inducer. However, this will only work if the tetraploid that you are trying to halve lacks the seed spot trait, as most modern, white potatoes do.
A Final Note on Probability
Anywhere that I have described a cross as impossible, that is not really true. Rather, that cross has a very low probability of success. There are circumstances under which almost every cross can work, sometimes yielding very odd results. It is true that triploids are a very unusual result for crosses between domesticated potato species, but it is also true that they sometimes occur. It only requires an unusual mutation or a failure of a key stage of meiosis for the supposedly impossible to occur. I have discussed some examples of known mechanisms for unusual compatibilities, but there are probably many more that we don’t know about. Because of the low probability, no professional would conceive a breeding program that relies upon such events, but that doesn’t mean that you won’t encounter one by accident or even that a dedicated amateur won’t achieve success by making non-viable crosses until one proves to be viable. In short, let this information guide you when you wish to make the most efficient use of your time, but don’t let it stop you if you are determined to achieve something unusual. The probability may be low, but it probably isn’t zero.
Determining Ploidy
Now that you know how you can use potato ploidy to your benefit, all that you need to do is to determine the ploidies of the varieties that you are working with. In most cases, this is pretty easy. In others, it might take some work. If you are working with common commercial potatoes in North America or Europe, they are almost certainly tetraploid. On the other hand, if you are working with experimental or seed-grown potatoes or you have made crosses that have unpredictable outcomes, you might need to investigate further. There are three reasonably reliable ways to determine ploidy, least to most reliable, but also easiest to hardest: 1. Morphological evaluation, 2. Guard cell counting, 3. Chromosome counting.
Morphological Evaluation
While it isn’t the most reliable way to determine ploidy, this is what most people rely on. It has the advantage of requiring no equipment, but even people with a lot of experience will be fooled some of the time. When evaluating complex tetraploid/diploid or wild potato crosses, I’ve found that I get it wrong or at least don’t feel sure about 10% of the time.
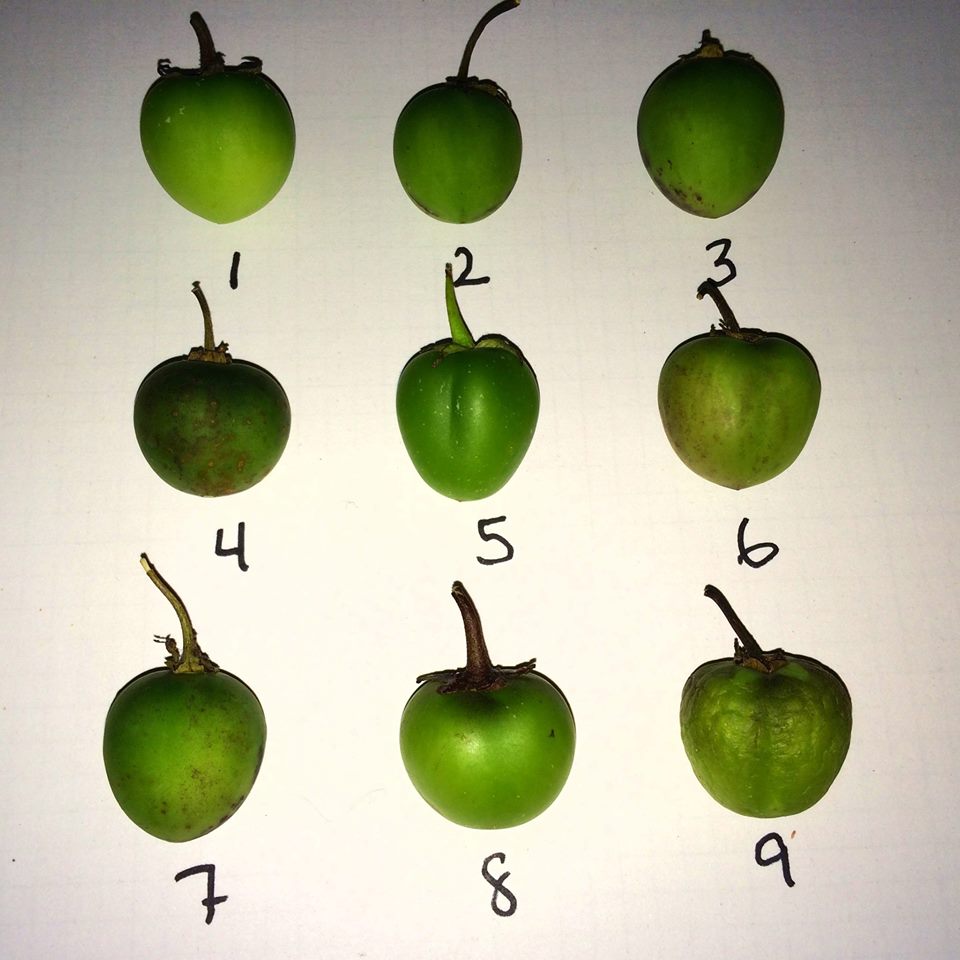
To determine tetraploids from diploids, you primarily want to look at the leaves and the berries.
Diploids will typically have long, thin leaves. The berries will often be tapered toward the bottom, with a sort of acorn shape.
Tetraploids will typically have rounder leaves, although there is considerable variation and it may be difficult to make a determination in leaf shape alone. The berries are generally very round, although all bets are off if you are dealing with a tetraploid that has a wild potato ancestor.
In general, if the leaves are long and thin and the berries are tapered, there is a very good chance that the potato is diploid. If the leaves are rather broad and the berries are round, there is a very good chance that it is tetraploid.
I have seen suggestions that the size of the plant, the size of the berries, and the thickness of the stem can aid in identification, but that isn’t true in my experience. Maybe if you have a very experienced eye.
Guard Cell Counts
This is probably the best compromise between reliability and accessibility. You need a microscope for this, but not a fancy one. 100x magnification is sufficient.
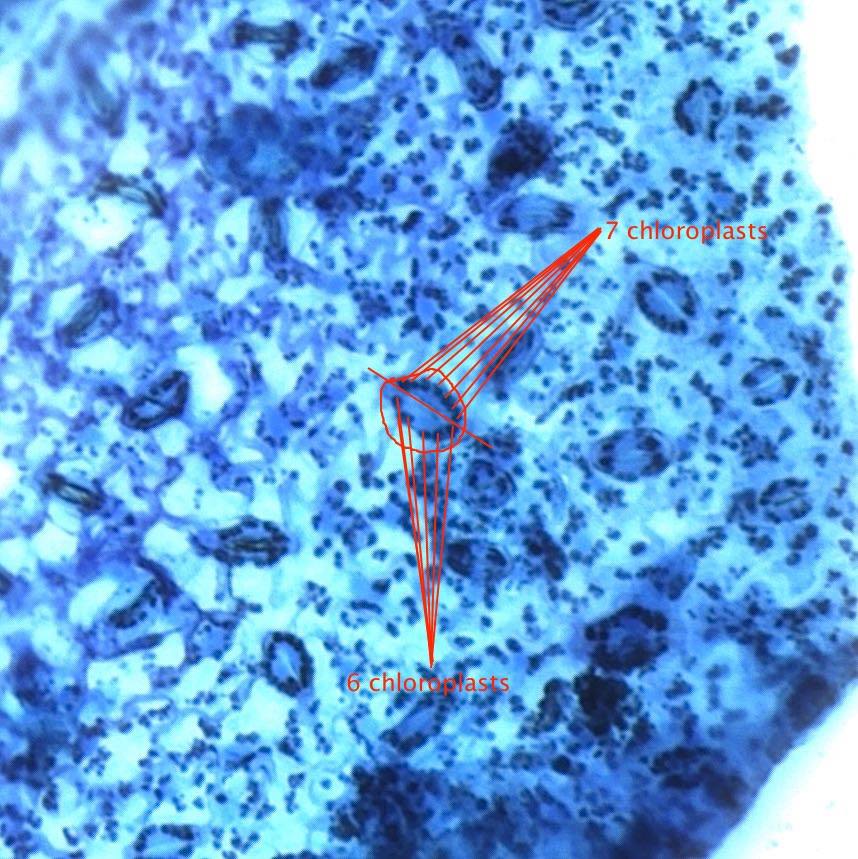
You need a microscope, a slide, and a bottle of Lugol’s iodine solution.
Bend a potato leaf in half and then pull it apart, trying to separate just the clear epidermis from the underside of the leaf. This will take a little practice. Ideally, you want a piece that is at least 1/3″ square.
Place the piece of epidermis on the slide and apply a drop of Lugol’s iodine. After about a minute, use a paper towel to draw off the excess iodine solution.
Place the slide under the microscope. You don’t need a cover slip, but be careful not to bring the objective in contact with the slide. Center the section using the lowest power objective and then move up to 100x (usually the second objective). You should be able to locate stomata, using the picture to the right as a guide. Within each stomatum, there are two guard cells, each of which contains a number of chloroplasts. Scan around until you find a region where you can clearly count the number of chloroplasts in at least 10 guard cells. Average the count.
Chloroplasts per Guard Cell | Chloroplasts per Stomatum | Probable Ploidy |
6-8 | 12-17 | Diploid |
9-11 | 18-23 | Triploid |
12-14 | 24-28 | Tetraploid (rarely Pentaploid) |
Chromosome Counting
This is the most certain method for determining potato ploidy, but you need an excellent microscope and good microscopy skills.
Allow a potato tuber to sprout. Remove the sprout and then grow it in water with a couple of drops of bleach until you can see rootlets forming at the base. Using a scalpel, remove a 1mm length of tip from a rootlet.
Place the root tip in a test tube with a few drops of 0.5% ocein stain solution and incubate at 80F for 48 hours.
Transfer the root tip to a slide and add a drop of the stain solution. Apply a cover slip and carefully press down to squash the root tip.
Locate cells that are undergoing mitosis and work up to your oil immersion objective. You should now be able to see cells in different stages of mitosis. You want to find cells that are in metaphase, which will allow you to count the chromosomes. If you aren’t familiar with the stages of mitosis, just do a search on the web. This is a staple of high school and college intro biology classes, so you will find plenty of information and pictures. You may have to scan around quite a bit to find cells that have countable chromosomes. Ideally, you should count at least ten cells and average the results. You should come very close to a multiple of 12, indicating the ploidy of the variety.
More Information
If you found this information useful, then you would probably also like the CIP Technical Manual of Potato Reproductive and Cytological Biology, which contains procedures for determining ploidy, pollen viability, and pollen germination.
I found this article extremely useful. My favorite quote “endosperm ratio is wonderful.” Thank you for helping me exercise ploidy determination.
I read this out of curiosity. You managed to make a heavy subject readable, highly appreciated.
I do plan on breeding diploid potatoes but don’t have any plans of messing with ploidity, this might still be good to know I guess.